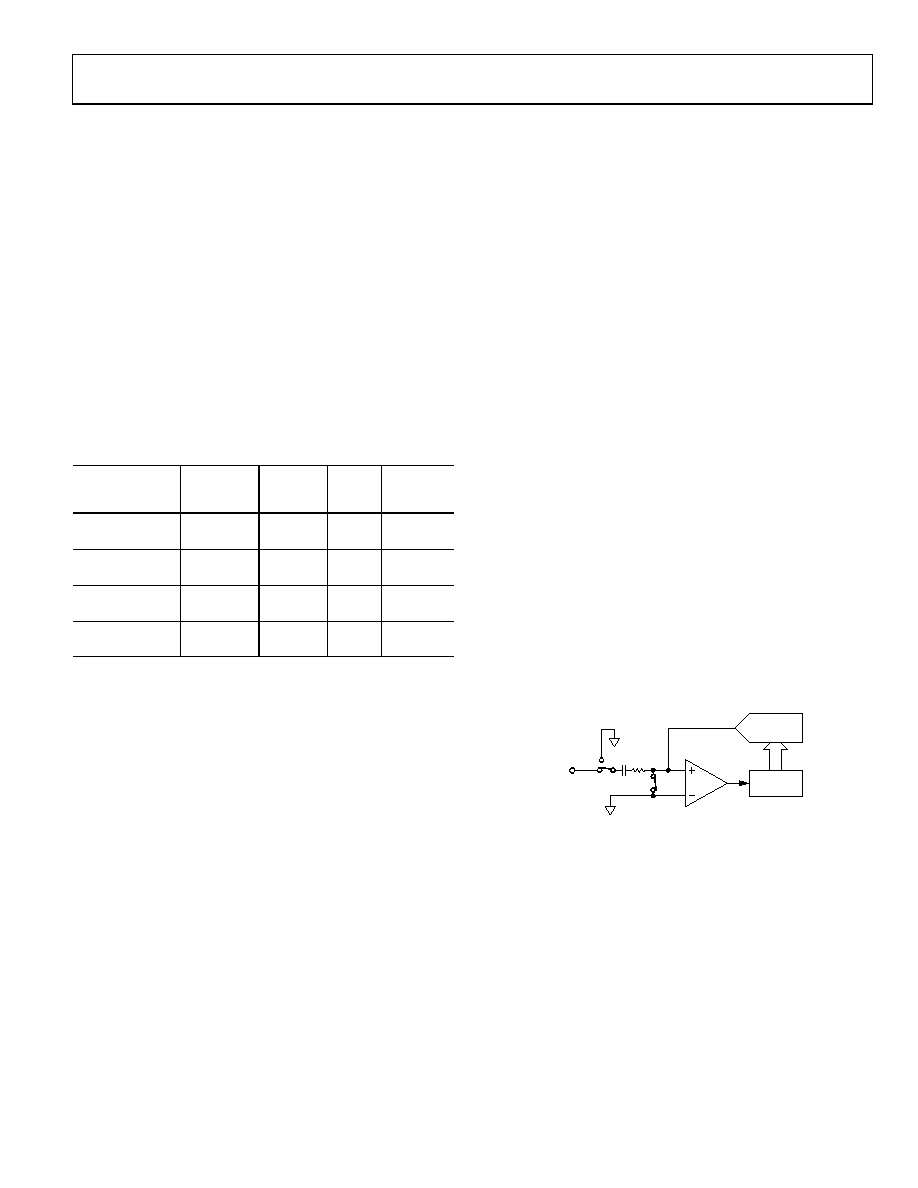
Data Sheet
AD7328
Rev. C | Page 15 of 36
THEORY OF OPERATION
CIRCUIT INFORMATION
Th
e AD7328 is a fast, 8-channel, 12-bit plus sign, bipolar input,
serial ADC. The
AD7328 can accept bipolar input ranges that
include ±10 V, ±5 V, and ±2.5 V; it can also accept a 0 V to +10 V
unipolar input range. A different analog input range can be
programmed on each analog input channel via the on-chip
registers. Th
e AD7328 has a high speed serial interface that
can operate at throughput rates up to 1 MSPS.
The AD7328 requires VDD and VSS dual supplies for the high voltage analog input structures. These supplies must be equal
to or greater than the analog input range. See Table 6 for the
requirements of these supplies for each analog input range. The
AD7328 requires a low voltage 2.7 V to 5.25 V VCC supply to power the ADC core.
Table 6. Reference and Supply Requirements for Each
Analog Input Range
Selected Analog
Input Range (V)
Reference
Voltage (V)
Full-Scale
Input
Range (V)
VCC (V)
Minimum
VDD/VSS
±10
2.5
±10
3/5
±10
3.0
±12
3/5
±12
±5
2.5
±5
3/5
±5
3.0
±6
3/5
±6
±2.5
2.5
±2.5
3/5
±5
3.0
±3
3/5
±5
0 to +10
2.5
0 to +10
3/5
+10/AGND
3.0
0 to +12
3/5
+12/AGND
1
Guaranteed performance for VDD = 12 V to 16.5 V and VSS = 12 V to 16.5 V.
The performance specifications are guaranteed for VDD = 12 V
to 16.5 V and VSS = 12 V to 16.5 V. With VDD and VSS supplies
outside this range, t
he AD7328 is fully functional but performance
is not guaranteed. When the
AD7328 is configured with the
minimum VDD and VSS supplies for a chosen analog input range,
the throughput rate should be decreased from the maximum
DNL as the VDD and VSS voltages are varied. When operating at
the maximum throughput rate, as the VDD and VSS supply voltages
are reduced, the INL and DNL error increases. However, as the
throughput rate is reduced with the minimum VDD and VSS
supplies, the INL and DNL error is reduced.
Figure 31 shows the change in THD as the VDD and VSS supplies are reduced. At the maximum throughput rate, the THD degrades
significantly as VDD and VSS are reduced. It is therefore necessary to
reduce the throughput rate when using minimum VDD and VSS
supplies so that there is less degradation of THD and the specified
performance can be maintained. The degradation is due to an
increase in the on resistance of the input multiplexer when the
VDD and VSS supplies are reduced.
The analog inputs can be configured as eight single-ended inputs,
four true differential input pairs, four pseudo differential inputs,
or seven pseudo differential inputs. Selection can be made by
programming the mode bits, Mode 0 and Mode 1, in the control
register.
The serial clock input accesses data from the part and provides the
clock source for the successive approximation ADC. Th
e AD7328has an on-chip 2.5 V reference. However, the
AD7328 can also
work with an external reference. On power-up, the external
reference operation is the default option. If the internal reference
is preferable, the user must write to the reference bit in the
control register to select the internal reference operation.
The
AD7328 also features power-down options to allow power
savings between conversions. The power-down modes are selected
by programming the on-chip control register as described in the
CONVERTER OPERATION
The
AD7328 is a successive approximation analog-to-digital
converter built around two capacitive DACs.
Figure 23 and
Figure 24 show simplified schematics of the ADC in single-
ended mode during the acquisition and conversion phases,
of the ADC in differential mode during acquisition and conversion
phases, respectively.
The ADC is composed of control logic, a SAR, and capacitive
DACs. I
n Figure 23 (the acquisition phase), SW2 is closed and
SW1 is in Position A, the comparator is held in a balanced con-
dition, and the sampling capacitor array acquires the signal on
the input.
CAPACITIVE
DAC
CONTROL
LOGIC
COMPARATOR
AGND
SW2
SW1
A
B
CS
VIN0
04852-
017
Figure 23. ADC Configuration During Acquisition Phase, Single-Ended Mode
When the ADC starts a conversion (see
Figure 24), SW2 opens and
SW1 moves to Position B, causing the comparator to become
unbalanced. The control logic and the charge redistribution
DAC are used to add and subtract fixed amounts of charge from
the capacitive DAC to bring the comparator back into a balanced
condition. When the comparator is rebalanced, the conversion
is complete. The control logic generates the ADC output code.