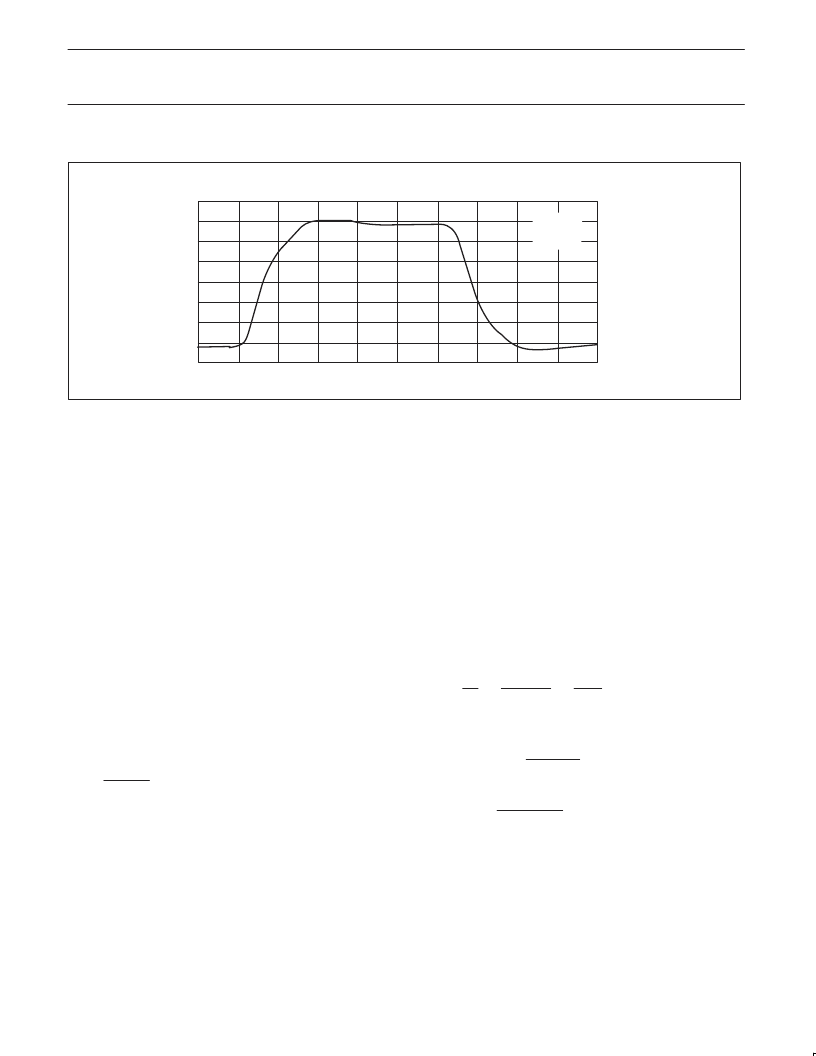
Philips Semiconductors
Product specification
SA5211
Transimpedance amplifier (180MHz)
1998 Oct 07
13
TYPICAL PERFORMANCE CHARACTERISTICS
(Continued)
0
2
4
6
8
(10
12
14
16
18
20
V
CC
= 5V
T
= 25
°
C
20mV/Div
Output Step Response
SD00334
Figure 10. Typical Performance Characteristics (cont.)
THEORY OF OPERATION
Transimpedance amplifiers have been widely used as the
preamplifier in fiber-optic receivers. The SA5211 is a wide bandwidth
(typically 180MHz) transimpedance amplifier designed primarily for
input currents requiring a large dynamic range, such as those
produced by a laser diode. The maximum input current before
output stage clipping occurs at typically 50
μ
A. The SA5211 is a
bipolar transimpedance amplifier which is current driven at the input
and generates a differential voltage signal at the outputs. The
forward transfer function is therefore a ratio of the differential output
voltage to a given input current with the dimensions of ohms. The
main feature of this amplifier is a wideband, low-noise input stage
which is desensitized to photodiode capacitance variations. When
connected to a photodiode of a few picoFarads, the frequency
response will not be degraded significantly. Except for the input
stage, the entire signal path is differential to provide improved
power-supply rejection and ease of interface to ECL type circuitry. A
block diagram of the circuit is shown in Figure 11. The input stage
(A1) employs shunt-series feedback to stabilize the current gain of
the amplifier. The transresistance of the amplifier from the current
source to the emitter of Q
3
is approximately the value of the
feedback resistor, R
F
=14.4k
. The gain from the second stage (A2)
and emitter followers (A3 and A4) is about two. Therefore, the
differential transresistance of the entire amplifier, R
T
is
R
T
V
OUT
(diff)
I
IN
2R
F
2(14.4K)
28.8k
The single-ended transresistance of the amplifier is typically 14.4k
.
The simplified schematic in Figure 12 shows how an input current is
converted to a differential output voltage. The amplifier has a
single input for current which is referenced to Ground 1. An input
current from a laser diode, for example, will be converted into a
voltage by the feedback resistor R
F
. The transistor Q1 provides most
of the open loop gain of the circuit, A
VOL
≈
70. The emitter follower Q
2
minimizes loading on Q
1
. The transistor Q
4
, resistor R
7
, and V
B1
provide level shifting and interface with the Q
15
– Q
16
differential
pair of the second stage which is biased with an internal reference,
V
B2
. The differential outputs are derived from emitter followers Q
11
–
Q
12
which are biased by constant current sources. The collectors of
Q
11
– Q
12
are bonded to an external pin, V
CC2
, in order to reduce
the feedback to the input stage. The output impedance is about 17
single-ended. For ease of performance evaluation, a 33
resistor is
used in series with each output to match to a 50
test system.
BANDWIDTH CALCULATIONS
The input stage, shown in Figure 13, employs shunt-series feedback
to stabilize the current gain of the amplifier. A simplified analysis can
determine the performance of the amplifier. The equivalent input
capacitance, C
IN
, in parallel with the source, I
S
, is approximately
7.5pF, assuming that C
S
=0 where C
S
is the external source
capacitance.
Since the input is driven by a current source the input must have a
low input resistance. The input resistance, R
IN
, is the ratio of the
incremental input voltage, V
IN
, to the corresponding input current, I
IN
and can be calculated as:
V
IN
I
IN
1
A
VOL
71
More exact calculations would yield a higher value of 200
.
R
IN
R
F
14.4K
203
Thus C
IN
and R
IN
will form the dominant pole of the entire amplifier;
1
2
R
IN
C
IN
Assuming typical values for R
F
= 14.4k
, R
IN
= 200
, C
IN
= 4pF
1
2
4pF 200
f
3dB
f
3dB
200MHz
The operating point of Q1, Figure 12, has been optimized for the
lowest current noise without introducing a second dominant pole in
the pass-band. All poles associated with subsequent stages have
been kept at sufficiently high enough frequencies to yield an overall
single pole response. Although wider bandwidths have been
achieved by using a cascade input stage configuration, the present
solution has the advantage of a very uniform, highly desensitized
frequency response because the Miller effect dominates over the
external photodiode and stray capacitances. For example, assuming
a source capacitance of 1pF, input stage voltage gain of 70, R
IN
=