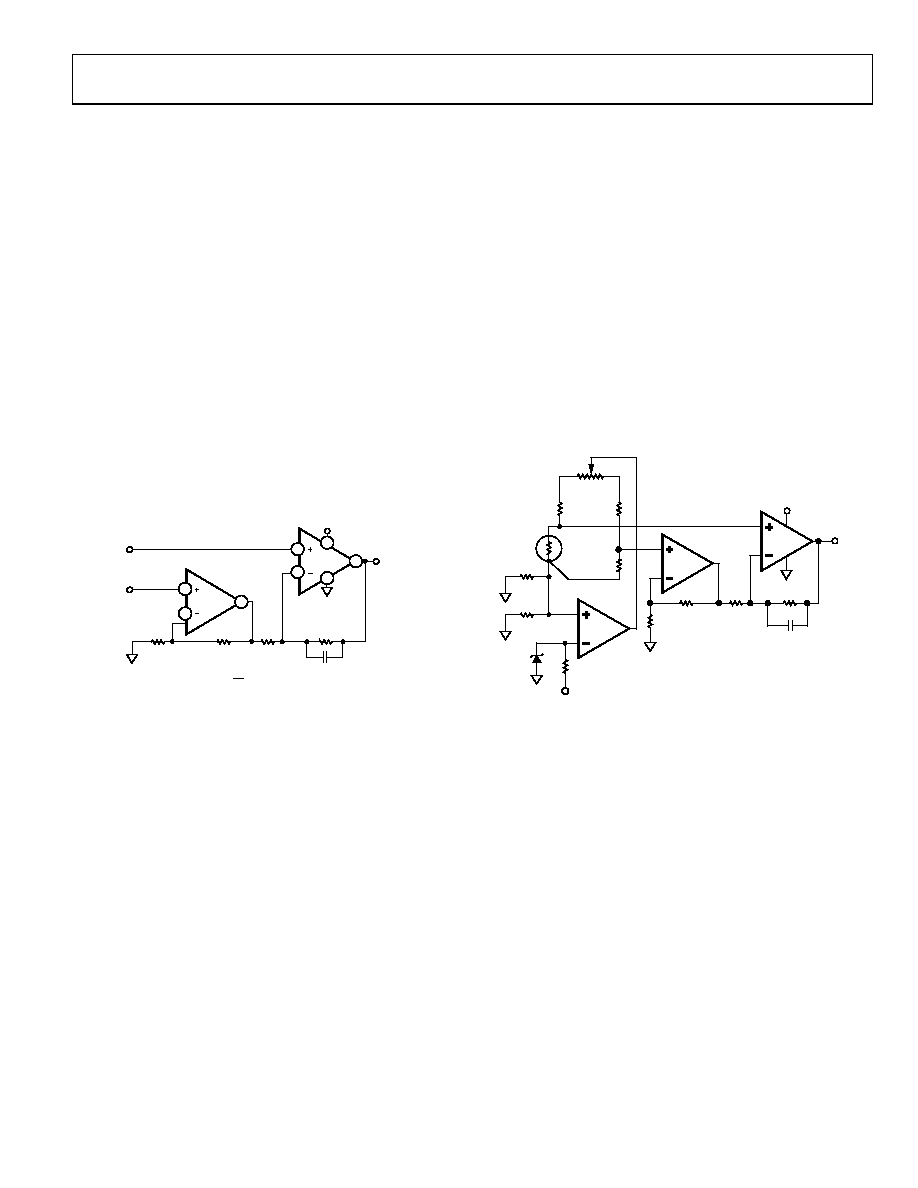
OP191/OP291/OP491
Rev. E | Page 19 of 24
APPLICATIONS INFORMATION
SINGLE 3 V SUPPLY, INSTRUMENTATION
AMPLIFIER
The OP291 low supply current and low voltage operation
make it ideal for battery-powered applications, such as the
instrumentation amplifier shown in
Figure 65. The circuit uses
the classic two op amp instrumentation amplifier topology, with
four resistors to set the gain. The equation is simply that of a
noninverting amplifier, as shown in
Figure 65. The two resistors
labeled R1 should be closely matched both to each other and to
the two resistors labeled R2 to ensure good common-mode
rejection performance. Resistor networks ensure the closest
matching as well as matched drifts for good temperature
stability. Capacitor C1 is included to limit the bandwidth and,
therefore, the noise in sensitive applications. The value of this
capacitor should be adjusted depending on the desired closed-
loop bandwidth of the instrumentation amplifier. The RC
combination creates a pole at a frequency equal to 1/(2π ×
R1C1). If AC-CMRR is critical, then a matched capacitor to C1
should be included across the second resistor labeled R1.
1/2
OP291
1/2
OP291
R1
R2
R1
3V
C1
100pF
VIN
VOUT
VOUT = (1 +
) = VIN
R1
R2
–
00
29
4-
0
69
3
2
1
8
5
6
4
7
+
Figure 65. Single 3 V Supply Instrumentation Amplifier
Because the OP291 accepts rail-to-rail inputs, the input
common-mode range includes both ground and the positive
supply of 3 V. Furthermore, the rail-to-rail output range ensures
the widest signal range possible and maximizes the dynamic
range of the system. Also, with its low supply current of
300 μA/device, this circuit consumes a quiescent current of
only 600 μA yet still exhibits a gain bandwidth of 3 MHz.
A question may arise about other instrumentation amplifier
topologies for single-supply applications. For example, a
variation on this topology adds a fifth resistor between the two
inverting inputs of the op amps for gain setting. While that
topology works well in dual-supply applications, it is inherently
for the traditional three op amp instrumentation amplifier. In
both cases, the circuits simply cannot work in single-supply
situations unless a false ground between the supplies is created.
SINGLE-SUPPLY RTD AMPLIFIER
The circuit in
Figure 66 uses three op amps of the OP491 to
develop a bridge configuration for an RTD amplifier that
operates from a single 5 V supply. The circuit takes advantage of
the OP491 wide output swing range to generate a high bridge
excitation voltage of 3.9 V. In fact, because of the rail-to-rail
output swing, this circuit works with supplies as low as 4.0 V.
Amplifier A1 servos the bridge to create a constant excitation
current in conjunction with the AD589, a 1.235 V precision
reference. The op amp maintains the reference voltage across
the parallel combination of the 6.19 kΩ and 2.55 MΩ resistors,
which generate a 200 μA current source. This current splits
evenly and flows through both halves of the bridge. Thus,
100 μA flows through the RTD to generate an output voltage
based on its resistance. A 3-wire RTD is used to balance the line
resistance in both 100 Ω legs of the bridge to improve accuracy.
1/4
OP491
VOUT
365
1/4
OP491
100k
0.01pF
A3
5V
GAIN = 274
100k
1/4
OP491
37.4k
5V
AD589
2.55M
6.19k
200
10 TURNS
26.7k
A2
A1
100
RTD
ALL RESISTORS 1% OR BETTER
00
29
4-
0
70
Figure 66. Single-Supply RTD Amplifier
Amplifier A2 and Amplifier A3 are configured in the two op
amp instrumentation amplifier topology described in the
Singlechosen to produce a gain of 274, such that each 1°C increase in
temperature results in a 10 mV change in the output voltage, for
ease of measurement. A 0.01 μF capacitor is included in parallel
with the 100 kΩ resistor on Amplifier A3 to filter out any
unwanted noise from this high gain circuit. This particular RC
combination creates a pole at 1.6 kHz.