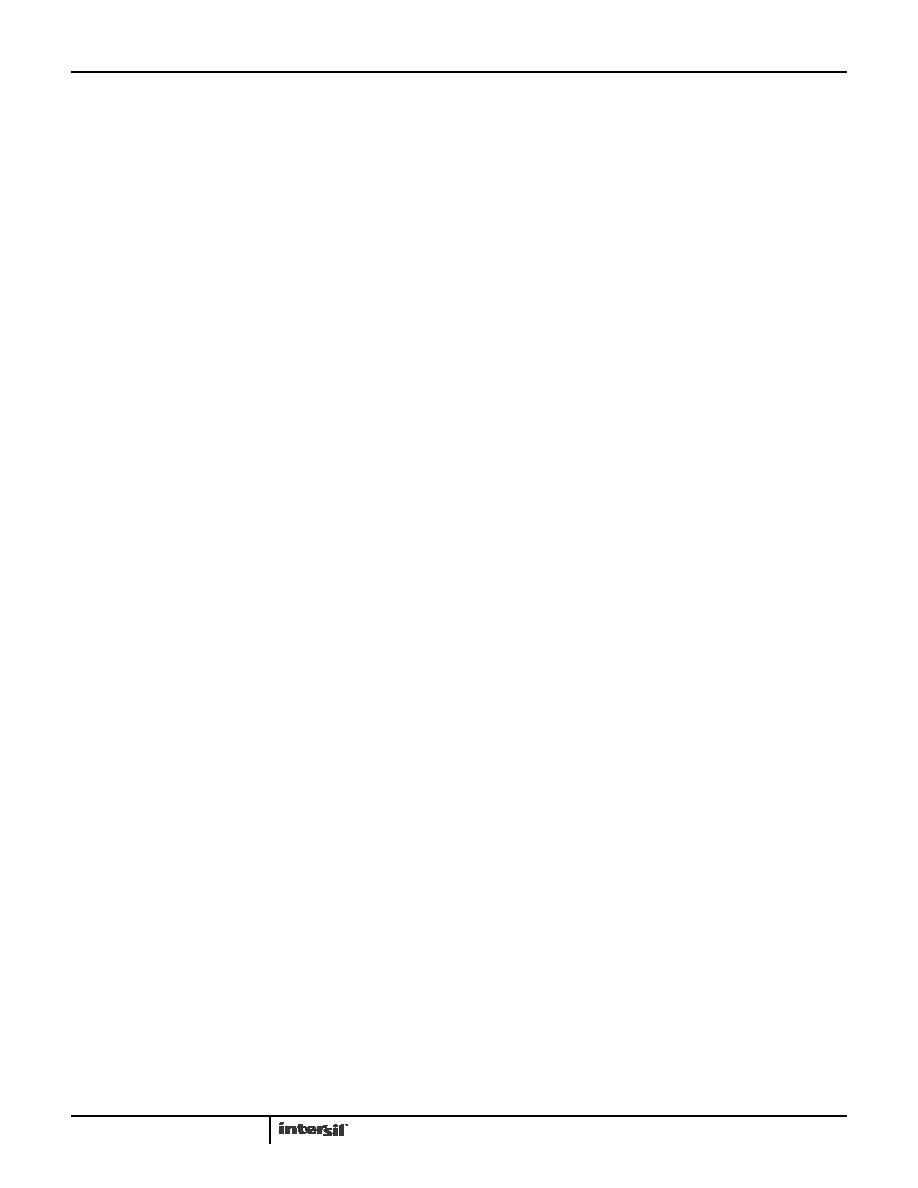
17
FN7177.2
March 31, 2011
Choice of Feedback Resistor, RF
These amplifiers are optimized for applications that require a
gain of +1. Hence, no feedback resistor is required.
However, for gains greater than +1, the feedback resistor
forms a pole with the input capacitance. As this pole
becomes larger, phase margin is reduced. This causes
ringing in the time domain and peaking in the frequency
domain. Therefore, RF has some maximum value that
should not be exceeded for optimum performance. If a large
value of RF must be used, a small capacitor in the few
picofarad range in parallel with RF can help to reduce this
ringing and peaking at the expense of reducing the
bandwidth.
As far as the output stage of the amplifier is concerned, RF +
RG appear in parallel with RL for gains other than +1. As this
combination gets smaller, the bandwidth falls off.
Consequently, RF also has a minimum value that should not
be exceeded for optimum performance.
For AV = +1, RF = 0惟 is optimum. For AV = -1 or +2 (noise
gain of 2), optimum response is obtained with RF between
300
惟 and 1k惟. For AV = -4 or +5 (noise gain of 5), keep RF
between 300
惟 and 15k惟.
Video Performance
For good video signal integrity, an amplifier is required to
maintain the same output impedance and the same
frequency response as DC levels are changed at the output.
This can be difficult when driving a standard video load of
150
惟, because of the change in output current with DC level.
(Differential Gain and Differential Phase curves for various
supply and loading conditions) will help you obtain optimal
performance. Curves are provided for AV = +1 and +2, and
RL = 150惟 and 10k惟 tied both to ground as well as 2.5V. As
with all video amplifiers, there is a common mode sweet spot
for optimum differential gain/differential phase. For example,
with AV = +2 and RL =150惟 tied to 2.5V, and the output
common mode voltage kept between 0.8V and 3.2V, dG/dP
is a very low 0.1%/0.1掳. This condition corresponds to
driving an AC-coupled, double terminated 75
惟 coaxial cable.
With AV = +1, RL = 150惟 tied to ground, and the video level
kept between 0.85V and 2.95V, these amplifiers provide
dG/dP performance of 0.05%/0.20掳. This condition is
representative of using the EL5144 series amplifier as a
buffer driving a DC coupled, double terminated, 75
惟 coaxial
cable. Driving high impedance loads, such as signals on
computer video cards, gives similar or better dG/dP
performance as driving cables.
Driving Cables and Capacitive Loads
The EL5144 series amplifiers can drive 50pF loads in
parallel with 150
惟 with 4dB of peaking and 100pF with 7dB
of peaking. If less peaking is desired in these applications, a
small series resistor (usually between 5
惟 and 50惟) can be
placed in series with the output to eliminate most peaking.
However, this will obviously reduce the gain slightly. If your
gain is greater than 1, the gain resistor (RG) can then be
chosen to make up for any gain loss, which may be created
by this additional resistor at the output. Another method of
reducing peaking is to add a 鈥渟nubber鈥� circuit at the output.
A snubber is a resistor in a series with a capacitor, 150
惟 and
100pF being typical values. The advantage of a snubber is
that it does not draw DC load current.
When used as a cable driver, double termination is always
recommended for reflection-free performance. For those
applications, the back-termination series resistor will de-couple
the EL5144 series amplifier from the cable and allow extensive
capacitive drive. However, other applications may have high
capacitive loads without a back-termination resistor. Again, a
small series resistor at the output can reduce peaking.
Disable/Power-Down
The EL5146 and EL5246 amplifiers can be disabled, placing
its output in a high-impedance state. Turn-off time is only
10ns and turn-on time is around 500ns. When disabled, the
amplifier鈥檚 supply current is reduced to 2.6A typically,
thereby effectively eliminating power consumption. The
amplifier鈥檚 power-down can be controlled by standard TTL or
CMOS signal levels at the CE pin. The applied logic signal is
relative to the GND pin. Letting the CE pin float will enable
the amplifier. Hence, the 8 Ld PDIP and 8 Ld SOIC single
amps are pin compatible with standard amplifiers that don鈥檛
have a power-down feature.
Short Circuit Current Limit
The EL5144 series amplifiers do not have internal short
circuit protection circuitry. Short circuit current of 90mA
sourcing and 65mA sinking typically will flow if the output is
trying to drive high or low but is shorted to half way between
the rails. If an output is shorted indefinitely, the power
dissipation could easily increase such that the part will be
destroyed. Maximum reliability is maintained if the output
current never exceeds
卤50mA. This limit is set by internal
metal interconnect limitations. Obviously, short circuit
conditions must not remain or the internal metal connections
will be destroyed.
Power Dissipation
With the high output drive capability of the EL5144 series
amplifiers, it is possible to exceed the +150掳C Absolute
Maximum junction temperature under certain load current
conditions. Therefore, it is important to calculate the maximum
junction temperature for the application to determine if load
conditions or package type need to be modified for the
amplifier to remain in the safe operating area.
The maximum power dissipation allowed in a package is
determined according to Equation
1:PDMAX
TJMAX - TAMAX
胃
JA
---------------------------------------------
=
(EQ. 1)
EL5144, EL5146, EL5244, EL5246, EL5444